The CAR T-cell manufacturing process involves a high potential for variability. Sensitive assays can help make sure these therapies strike the crucial balance of safety and efficacy with every batch.
Chimeric antigen receptor (CAR) T-cell therapies have given years back to patients with cancer for whom no other treatment worked. But as new CAR T-cell products proceed through preclinical development and clinical trials, each treatment — even batches of the same therapy — can vary significantly. Developers can produce CAR T-cell therapies in multiple ways. Furthermore, although each batch is created from the same patient’s T cells, they may grow and behave differently because of other treatments the individual previously received.
As a result, quality control and quality assurance are especially crucial in the CAR T-cell manufacturing process. Developers can use molecular methods to test CAR T-cell potency, persistence, and batch contamination. These quality control practices maximize the therapies’ potential to help patients while minimizing the risk of harm, which in turn makes it more likely that a given CAR T-cell treatment will succeed in clinical trials. To reap these quality control benefits, scientists need highly sensitive, accurate tools to monitor the clinical manufacturing of CAR T cells to make sure the process yields an end product that will be safe and effective when administered to every patient.
Using the Right Technology to Get the Right CAR T-Cell Dose
Depending on lab conditions, cell health, and other hard-to-predict factors, different batches of T cells take up varying numbers of CAR transgene copies upon transfection. CAR T cells that contain too many copies of the CAR transgene have the potential to trigger dangerous immune responses in patients’ bodies, but T cells that fail to take up any copies won’t attack cancer cells effectively (Brudno and Kochenderfer 2016). The FDA has stated that CAR T cells should contain between one and four transgene copies (a narrow range) (Zhao et al. 2017). As a result, developers need a highly precise way to determine the cells’ CAR transgene copy number to avoid dosing a patient with cells that have too many or too few copies.
Until recently, quantitative PCR (qPCR) has been the go-to method for transgene copy number determination in CAR T-cell quality control and many other areas. However, because of its reliance on a standard curve, this method is susceptible to human error and variability and cannot detect down to one gene copy per cell. Therefore, it is not accurate or precise enough to reliably tell researchers whether CAR T cells contain a safe and efficacious copy number of the CAR transgene. In contrast, Droplet Digital PCR (ddPCR) provides absolute quantification of nucleic acids and is, therefore, ideal for accurate transgene copy number determination (Lu et al. 2020).
Scientists have proven the superior sensitivity of ddPCR technology with rigorous laboratory testing. Yaoyao Lou, PhD, and her colleagues at the Huazhong University of Science and Technology in Wuhan, China compared qPCR and ddPCR assays for CAR T-cell quality control (Lou et al. 2020). The team found that their ddPCR assays were more sensitive than qPCR alternatives when measuring diluted CAR DNA standards, detecting down to 3.2 transgene copies per milliliter while qPCR could not detect such a low concentration.
How Long Do CAR T-Cell Products Persist in Patients’ Bodies?
Going beyond transgene copy number, researchers and clinicians need to determine and optimize CAR T-cell persistence — the length of time the altered cells remain alive and circulating in a patient’s body. Ideally, cells should persist for several months after treatment to ensure that the cancer is fully eradicated. However, CAR T cells that linger beyond that point can lead to side effects, such as adverse immune or neurologic events (Lou et al. 2020).
Researchers and medical professionals can monitor CAR T-cell persistence over time with serial ddPCR assays that detect and quantify CAR T cells in blood samples (Mika et al. 2020). Luo and her colleagues found that qPCR was only sensitive enough to detect down to 20 CAR copies per reaction in blood samples, whereas ddPCR technology could detect as few as five copies per reaction. The ddPCR assays also demonstrated superior reproducibility (Lou et al. 2020).
Detecting Replication-Competent Viruses and Other Contaminants
One of the other challenges of CAR T-cell therapy development is preventing batch contamination. The viral vectors used to transfect T cells with the CAR transgene could theoretically replicate and persist within the patient; if this ever occurred, it could have severe health consequences. To prevent this from happening, the FDA says scientists should test for replication-competent viruses throughout the clinical manufacturing of CAR T cells and in patients after administration (U.S. FDA 2001).
Because it is so sensitive, ddPCR technology has proven to be an ideal tool to detect even the smallest traces of replication-competent virus, allowing manufacturers to screen out any virus-containing batches before the cells are infused into patients (Wiltshire et al. 2021). Like other cell and gene therapies, CAR T-cell products are also susceptible to other problematic contaminants, such as Mycoplasma bacteria. ddPCR assays are the most sensitive tool for detecting these at each stage of the manufacturing process.
The Future of CAR T-Cell Therapy
CAR T-cell therapies will only continue to advance and become more diverse in the years to come. Researchers are already tackling an ever-widening variety of cancer types — including solid tumors — with new types of CAR T cells (Sterner and Sterner 2021). It stands to reason that developers will need quality control methods that can keep up with this evolving power and complexity.
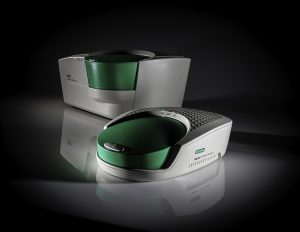
The QX200 Droplet Digital PCR System offers excellent precision and sensitivity.
ddPCR technology offers the sensitivity, precision, and versatility needed to accommodate these developments. By leveraging this technology and working with regulators to improve standards and methods for the CAR T-cell manufacturing process, biopharmaceutical industry leaders can bring more life-saving treatments into the clinic to benefit patients.
Visit the Bio-Rad website to see how Droplet Digital PCR technology can improve your CAR T-cell therapy quality control.
References
Brudno JN and Kochenderfer JN (2016). Toxicities of chimeric antigen receptor T cells: Recognition and management. Blood 127, 3,321–3,330.
Lou Y et al. (2020). Detection and quantification of chimeric antigen receptor transgene copy number by Droplet Digital PCR versus real-time PCR. J Mol Diagn 22, 699–707.
Lu A et al. (2020). Application of Droplet Digital PCR for the detection of vector copy number in clinical CAR/TCR T cell products. J Transl Med 18, 191.
Mika T et al. (2020). Digital-droplet PCR for quantification of CD19-directed CAR T-cells. Front in Mol Biosci 7, 84.
Sterner RC and Sterner RM (2021). CAR-T cell therapy: Current limitations and potential strategies. Blood Cancer J 11, 69.
U.S. FDA (2001). Supplemental guidance on testing for replication-competent retrovirus in retroviral vector-based gene therapy products and during follow-up of patients in clinical trials using retroviral vectors. Hum Gene Ther 12, 315–320.
Wiltshire T et al. (2021). Sensitive detection of integrated and free transcripts in chimeric antigen receptor T-cell manufactured cell products using Droplet Digital polymerase chain reaction. Cytotherapy 23, 452–458.
Zhao Y et al. (2017). Development of the first World Health Organization lentiviral vector standard: Toward the production control and standardization of lentivirus-based gene therapy products. Hum Gene Ther Methods 28, 205–214.