Use of IMAC at Process Scale
Selection of an IMAC resin with the most efficient features is critical for process protein purification. A resin with a large bead size (>90 µm) might not generate high back pressure at the required flow rates, but runs the risk of having a low dynamic binding capacity (DBC) (Figure 1), which is not ideal for process purification. A resin with a small bead size (<35 µm) might have a relatively higher DBC (Figure 1), but can run the risk of generating high back pressures at the flow rates required for process purification. This can be detrimental, as high back pressures can compromise the integrity of the column. Process-scale purifications require a resin with an optimized bead size that retains a high DBC while allowing high flow rates, without generating excessive back pressure. The Nuvia™ IMAC Resin incorporates these features. We performed a flow rate vs. DBC analysis on Nuvia IMAC and four commercially available IMAC resins. Nuvia IMAC provided consistently high DBC (>40 mg/mL), even at flow rates of 300 cm/hr (Figure 1), which ensures a quick, time-efficient purification, making Nuvia IMAC suitable for process purification.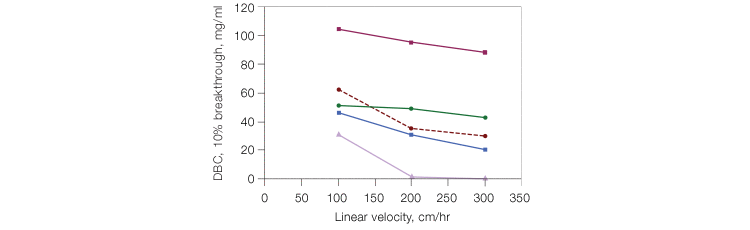
Fig. 1. Dynamic binding capacity vs. flow velocity of Nuvia IMAC Resin. Comparison of DBCs between Nuvia IMAC and two other commercially available IMACs. The resins were packed into 1 ml columns (0.5 x 5 cm). Histidine-tagged green fluorescent protein (GFP) (1.2 mg/ml) in 50 mM sodium phosphate, 5 mM imidazole, and 300 mM NaCl, pH 7.5, was loaded onto each column until 10% breakthrough (BT) was observed. Nuvia IMAC, 50 µm (●); Resin 1, 34 µm (■); Resin 2, 45−165 µm (▲); Resin 3, 60−160 µm (■); Resin 4, 45−165 µm (●).
In comparison, most of the other resins tested showed decreasing DBC with increasing flow rates. Resin 1 showed higher DBC than Nuvia IMAC, most likely due to its small pore size. However, the potential for an increase in back pressure with high flow rates has to be considered during process purification. In order to estimate if the back pressure generated in Nuvia IMAC under high flow rates was within the acceptable range for process purification, we performed a pressure flow curve with a histidine-tagged protein. The column pressure remained below 2 bar at a linear velocity of 300 cm/hr (Figure 2), indicating that Nuvia IMAC can be used at process-scale levels. In addition, Nuvia IMAC resin showed no significant loss in its DBC over more than 100 cycles of repeated use (Figure 3), making it a cost-effective option.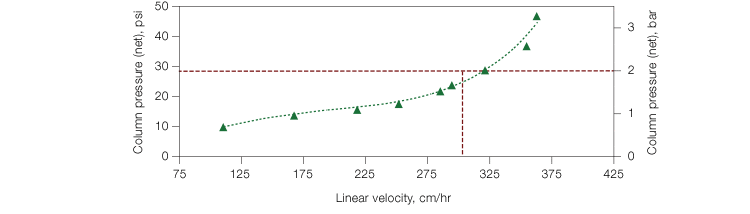
Fig. 2. Pressure/flow performance of Nuvia IMAC Resin. Uncharged Nuvia IMAC Resin slurry prepared in water was packed into a 20 x 20 cm column by axial compression with a compression factor of 1.2.
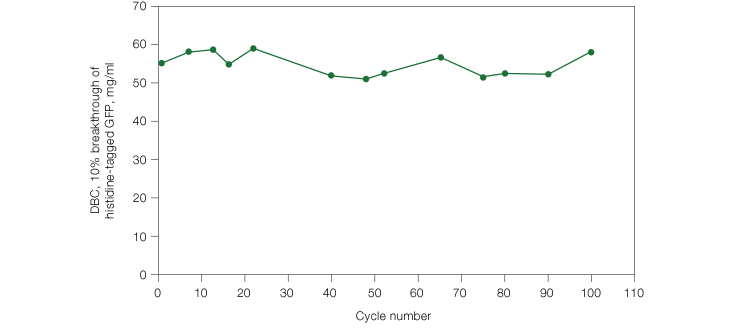
Fig. 3. Reusability of Nuvia IMAC Resin. Histidine-tagged GFP was loaded onto a 0.5 x 5.1 cm column packed with Nuvia IMAC with a compression factor of 1.25. The column was run at 300 cm/hr. The binding buffer was 50 mM sodium phosphate, 5 mM imidazole, and 300 mM NaCl, pH 7.5, and the elution buffer contained 250 mm imidazole. The column was stripped with 50 mM EDTA, pH 8.0, cleaned with I N NaOH, and recharged with 0.1 M Ni2SO4.
Typically, depending on the column size, the column performance can vary, despite similar packing. This warrants an upfront investment of cost and time to optimize the process-scale column packing for the most efficient column performance. Nuvia IMAC shows reproducibility in packing efficiency at small- and large-scale in terms of asymmetry (As) and reduced height equivalent of theoretical plate (rHETP), as shown in Table 1. This enhances the efficiency of the purification.
Column Size | As | rHETP |
1.6 x 20 cma | 0.9–1.2 | 2.0–2.4 |
10 x 20 cma | 0.9–1.0 | 2.4–3.1 |
20 x 20 cmb | 0.8–1.2 | 1.8–2.2 |
Table 1. Packed column performance. Nuvia IMAC was packed in three different-sized columns and the performance of the columns was tested by performing asymmetry (As) and reduced HETP analysis (rHETP) analysis. a: flow pack, b: axial compression.
Histidine-Tagged Purification Process
We used Nuvia IMAC in a purification process devised for therapeutic proteins with the intent of purifying a protein with minimal endotoxin and leached nickel levels. Endotoxin levels were tested using a LAL assay and nickel levels were quantitated by ICP-MS analysis (data not shown). Capture purification. A recombinant histidine-tagged protein was loaded onto a column packed with Nuvia IMAC. The protein was eluted in a bind-elute mode with imidazole. The eluted protein was >90% pure. Intermediate purification. The intermediate purification was performed on Bio-Rad’s Nuvia™ cPrime™ Mixed-Mode Resin. This resin combines hydrophobic interaction and cation exchange chromatography capabilities (HIC and CEX, respectively). The eluate from the capture step was loaded and washed and the protein was collected in flow-through mode. Sample analysis showed decreased host cell protein (HCP) contamination. In addition, decreased levels of endotoxin content and leached nickel from the prior step were also seen (Table 2). Polish purification. Polish purification was performed on CHT™ Ceramic Hydroxyapatite Mixed-Mode Media. This media combines affinity interaction and CEX capabilities. The pretreated eluate from the previous step was loaded onto the CHT Column. The protein was extracted in bind-elute mode. Analysis of the eluted samples showed negligible endotoxin and leached nickel (Table 2).Step | Resin Used | Endotoxin (EU/mg)a | Ni2+ (μg/g)b |
E. coli extract | — | 1.02 x 105 | — |
Capture | Nuvia IMAC | 5.13 x 103 | 1.4 |
Intermediate | Nuvia cPrime | 3.95 x 103 | 0.12 |
Polish | CHT | 3.98 x 102 | 0.008 |
Table 2. Comparison of histidine-tagged protein purification performance. The protein was purified with three different resins, and the levels of endotoxin and leached Ni were analyzed. a: LAL assay, per milligram of protein; b: ICP-MS analysis, per gram of solution. Detection limit: 0.002 mg/g.
Conclusions
IMAC purification for histidine-tagged proteins offers numerous advantages including low costs, simplicity of use, and robust purification efficiencies. Nuvia IMAC Resin offers these features, in addition to simple scalability. This enhances the efficiency of the purification process and makes Nuvia IMAC an ideal resin for process purification. This resin is also compatible with a broad array of chemicals and exhibits stability in the presence of commonly used protein purification reagents.First posted on genengnews.com on May 1, 2017.