The same type of materials that make up a nylon windbreaker may one day block HIV infection, thanks to new techniques in synthetic chemistry. The materials in question are polymers, the large molecule chains that, in their man-made forms, go into everything from Styrofoam cups to airplane wings. Dan Mitchell is a researcher at the University of Warwick, UK, who is studying how to use these synthetic polymers in biological settings such as the human immune system.
The key in making polymers that can interact in biological processes lies in better control of the polymers’ molecular sequences, Mitchell says. “Over the last ten or fifteen years,” he explains, “The types of chemical reactions used to synthesize polymers have elaborated, so you can now develop polymers of a highly defined length and size.”
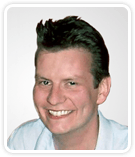
RCUK Academic Fellow at Warwick Medical School
University of Warwick, UK
Mitchell and his research colleagues wanted to use these new synthesis techniques to make polymers that would interact with a specific receptor, the carbohydrate binding protein DC-SIGN, found on human dendritic cells that move through the body like beat cops on patrol for the immune system. “DC-SIGN is an interesting protein,” Mitchell says, “because this protein is present in all people, which suggests that it’s fundamental to human health and development.”
Along with being universal among humans, DC-SIGN interested Mitchell and his colleagues for the key role it plays in HIV infection. In order to take over the CD4 cells and begin replicating en masse, ultimately overwhelming the immune system, HIV virions need to travel from the initial infection site deeper into the body to the lymph nodes. They do this by attaching to roving peripheral dendritic cells, binding to DC-SIGN receptors using what Mitchell describes as their “sugar-coating,” (van den Berg, Geijtenbeek, 2013) specially evolved glycoproteins on their viral envelope.
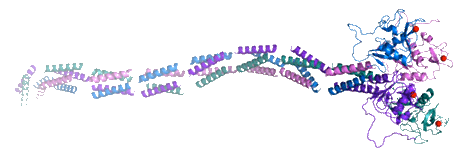
Structure of DC-SIGN protein. One link in the chain of HIV infection occurs when the virus binds to dendritic cells, which transport the pathogen to the lymph nodes where it begins to take over CD4 cells. The DC-SIGN receptor on the dendritic cells is one known binding site for the virus and was the target of interest for Mitchell and colleagues’ glycopolymers.
Making polymers that would bind to DC-SIGN could inhibit this HIV infection process, potentially acting as a microbicide by stopping the virus from attaching to dendritic cells and reaching the CD4 cells. DC-SIGN’s affinity for the HIV envelope glycoprotein gp120 also served as a known quantity for testing Mitchell’s novel glycopolymers.
Using techniques fully described in their recent paper (Zhang et al. 2013), Mitchell and his colleagues achieved a high degree of control over the structure of the polymers they synthesized. “Unlike mixing lots of different polymer lengths together as you would to make a composite like nylon fabric,” Mitchell explains, “we made polymers that were sequence-contolled at the molecular level.”
Thanks to this new degree of control, Mitchell and his colleagues now had several classes of molecules with well-defined structures they could then test in various interactions. “We had a range of molecules with different types of carbohydrate molecules at distinct points along the polymer backbone,” he says. “We wanted to see which ones bound to DC-SIGN better or worse, and did that correlate with the existence of sugars at specific points in those polymers?”
To test the interactions of these novel glycopolymers, Mitchell and his colleagues turned to Bio-Rad’s ProteOn™ XPR36 protein interaction array system. This instrument utilizes surface plasmon resonance (SPR) to enable highly multiplexed analysis of different unlabeled molecules in real time. By flowing up to six analytes in solution phase through microfluidic channels across up to six ligands immobilized on a gold sensor chip, the ProteOn system can analyze up to 36 different interactions in one run. SPR responses at the sensor surface over the course of the run are proportional to the binding level of analytes to ligands, producing a sensorgram that, when analyzed using a suitable model, results in a quantitative measurement of molecular affinity.
“The ProteOn system has been absolutely indispensable in getting quantitative data on the interactions between the glycopolymers and DC-SIGN,” says Mitchell. “It’s been central to the characterization of these polymers at the biological level.”
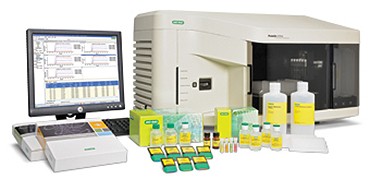
The ProteOn XPR36 protein interaction array system enables researchers to obtain the kinetics of up to 36 different analyte-ligand binding interactions simultaneously.
In obtaining this characterization, Mitchell first passed different concentrations of the synthesized glycopolymers across immobilized DC-SIGN. “The numbers we got from the ProteOn system analysis were biologically relevant within the physiological range of high affinity,” he says. “It was important to have quantitative data on this because sometimes in the literature an affinity is claimed, but there’s no quantitative data to say how strong that binding is and what characteristics it possesses. Does it stick quickly and fall off quickly? Does it stick quickly and fall off slowly? These are very important pieces of chemical information that the ProteOn system provides.”
Along with getting high-quality quantitative data, Mitchell also appreciated the throughput capacity for the ProteOn system. “For this particular set of experiments, the ProteOn system is pretty much the best system you could think of,” he says. “Because of the sheer number of glycopolymers we were making, we only did them in runs that yielded only a few miligrams. From that pool of material, however, we could run dozens of experiments thanks to the operating capacity of the system. Using other methods, such as isothermal titration calorimetry, we would have spent six months to a year generating sufficient quantities of material for the experiment.”
Having established that his novel glycopolymers had various levels of affinity for DC-SIGN, Mitchell then proceeded to the most crucial question in his study: could these new polymers inhibit HIV, specifically the binding of the virus’s gp120 envelope to DC-SIGN?
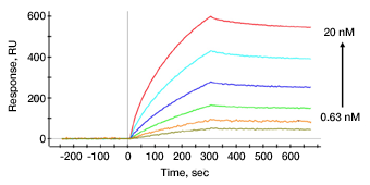
A sensorgram showing kinetic analysis of the novel glycopolymers with the DC-SIGN protein. Mitchell examined the glycopolymers’ binding interactions with the DC-SIGN protein, as well as their ability to compete for DC-SIGN with the HIV protein gp120.
To answer this question, Mitchell and his colleagues set up competition assays, this time flowing DC-SIGN and various combinations of the polymers over immobilized gp120. “Putting those polymers together with recombinant DC-SIGN protein in the SPR platform allowed us to analyze multiple polymers simultaneously,” Mitchell says. “We got a lot of data very quickly, and that allowed us to do comparisons between different classes of the polymers we were making.”
With these assays, Mitchell was able to see that some of the novel glycopolymers did indeed inhibit gp120 binding to DC-SIGN. In particular, those polymers that incorporate mannose sugars had the highest inhibitory values. Mitchell is excited to investigate these molecules further and sees several research avenues opening as a result of this finding.
“The next step is to use these polymers in experiments with very high quality cells or even tissues,” Mitchell says. “We need to get cells from human beings, incubate them with the polymers, and study their responses. We don’t have that set up yet.” He is currently reaching out to biomedical researchers to prepare this new round of experiments. After they better understand how the polymers interact with DC-SIGN in vitro, further studies could test them using more robust HIV models.
On another track, Mitchell wants to use the ProteOn system to test an even wider range of novel glycopolymers. “I’m guessing here, but there are about 30 to 40 carbohydrate-binding receptors on key cells such as dendritic cells or macrophages,” he notes. “With the right network of people there’s no reason why we can’t synthesize hundreds of different polymers that might target these receptors, and the ProteOn system would be central to this approach. It’s the perfect screening tool and it’s ideal for high-resolution characterization.”
Where might these new polymers — man-made materials that can interact biologically — ultimately lead in terms of applications? Mitchell returns to the importance and ubiquity of the DC-SIGN receptor as one example. While its role is still being studied, evidence suggests that DC-SIGN plays a key role in modulating immune response, part of a mechanism that holds the system back rather than activating a response. Polymers, Mitchell speculates, might provide further help in this process.
“If you have novel agents such as these polymers that can be produced in large quantities,” he says, “you could potentially target DC-SIGN as a means of modulating immune response. So my colleagues and I wonder if someday we might use polymers to, say, help transplant patients tolerate their new organs better? There could be many instances where you could use them beneficially, to just nudge the immune system in the right direction.”
References
van den Berg LM, Geijtenbeek TB. Antiviral immune responses by human langerhans cells and dendritic cells in HIV-1 infection. Advances in Experimental Medicine and Biology 762, 45-70.
Zhang Q et al. Sequence-controlled multi-block glycopolymers to inhibit DC-SIGN-gp120 binding. Angewandte Chemie International Edition 52(16), 4435-9.